Radiopharmaceuticals in the 21st Century
Posted on: Sunday 4 February 2024
Author: Dr C Gillies O’Bryan-Tear MB FRCP FFPM MBA and FPM's Oncology Expert Group.
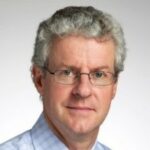
Introduction
Since the discovery of the X-ray by Wilhelm Roentgen in Germany in 1895 and subsequent work by Henri Becquerel and Marie and Pierre Curie on radioactive decay, radiation in medicine has become established in both diagnosis and treatment. These early pioneers gave their names to the units of measurement we still use to measure radiation, whether it is the amount released, the exposure, the amount absorbed, or the effective dose.
The medical use of ionising radiation, whether delivered externally or internally, is limited due to its adverse effects on healthy tissue. However, in this blog, I will summarise the recent advances in the field of targeted radiopharmaceuticals in oncology; the rapid growth of these agents has come with the advent of more sophisticated chelation and linker agents and a deepening understanding of how to deploy them.
Types of ionising radiation
In addition to X-rays, three other types of radiation are used in medicine: alpha, beta and gamma. These types of radiation are typically emitted from isotopes as part of their natural radioactive decay process towards a stable atom. An example is shown in Fig 1 [1].
Alpha particles are the heaviest type of particulate radiation, consisting of a positively charged (2+) helium nucleus (two protons and two neutrons); they have high energy and reliably cause double-stranded DNA breaks and cell killing; they require 1-5 hits to kill a cell (by comparison, beta emitters require 100-1,000). However, owing to their mass, they do not penetrate far, up to 100µM (ten cell lengths approximately). They are, therefore, highly toxic if ingested and distributed indiscriminately – for example, polonium-210, used to murder the Russian ex-spy Alexander Litvinenko in 2006. (Polonium was named after Poland as Marie Curie discovered it). External exposure is relatively harmless since the particles have such a short distance over which they act, making handling relatively easy. Recent research has focussed on the systemic administration of these potent agents to specific tumour types using small molecule or antibody targeting ligands; if well targeted, because of their short path length and high potency, they achieve higher therapeutic ratios than other types of radiation, but there do remain off target toxicity liabilities – for example, if excreted really. Figure 1 shows the decay cycle for 223Ra [1].
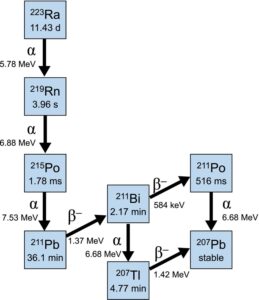
Beta particles are fast-moving electrons or positrons. Beta-emitting isotopes are widely used medically, e.g. 131 Iodine for thyroid disease. Beta particles are easily stopped by clothing or aluminium, but when targeted to tissues, they can produce useful therapeutic effects. For example, Pluvicto (177 Lu-PSMA-617), a prostate-specific membrane antigen (PSMA) targeted radiotherapeutic that has shown efficacy in late-stage castration-resistant prostate cancer, is now widely used. Older beta emitters include Zevalin (rituximab combined with 90Yttrium) and Bexaar (131Iodine tositumomab); both are CD20 targeted beta emitters for non-Hodgkin’s lymphoma, neither of which achieved widespread use or commercial success for various reasons.
Gamma rays are not particles but high-energy electromagnetic waves (even more energetic than X-rays). They have high penetrance and are released during the decay of various isotopes and by nuclear explosions. They are used for imaging to study the biodistribution of radiopharmaceuticals.
It is worth mentioning that positron-emitting glucose analogues such as 18Fludeoxyglucose (FDG) are widely used in cancer imaging. The glucose analogue is taken up more avidly in tumours which have high metabolic rates, where they release a positron. The interaction of that positron with a local electron results in the emission of gamma radiation that can be detected by imaging detectors (gamma cameras).
Figure 2 illustrates the differences in penetration between the different types of radiation [2].
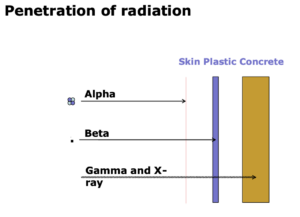
Many medically useful isotopes (naturally occurring or, more commonly, produced using cyclotrons) produce more than one type of radiation as they move through different stages of decay (Fig 1) [1]. The rate at which they produce this radiation as they decay towards a stable (non-radioactive) atom is defined by their half-life – this is a useful measure of both the “shelf life” of the product and the time the patient may need to keep isolated from others after administration.
Prostate cancer – a paradigm application for radiopharmaceuticals
Prostate cancer is treated initially by resection or local irradiation, with high cure rates. If it has spread beyond the capsule or distantly, additional treatment is needed, and secondaries often develop in the bone. Initially, these cancers are driven hormonally by androgens, so the patients are rendered castrate by luteinising hormone-releasing hormone (LHRH) and androgen receptor antagonists (originally, orchiectomy was used). When they escape from these androgen deprivation therapies (ADT), which they all do eventually, they become castration resistant (CRPC), and this group of patients has seen an explosion of effective therapies in the last decade. Docetaxel was the first chemotherapy approved in this setting and demonstrated significant survival improvement [3].
The next wave of therapies were androgen synthesis and androgen receptor inhibitors (androgen receptor pathway inhibitors, ARPI’s), which achieve more profound levels of cellular androgen inhibition than LHRH and block the continuing intracellular androgen agonism, which drives tumour progression even after chemical castration. These new therapies (for example, abiraterone and enzalutamide) have transformed the treatment of late-stage prostate cancer in the last decade, achieving many months or years of prolonged good quality life and being used earlier in the treatment pathway. Abiraterone was developed at the Royal Marsden Hospital in the UK.
Xofigo
Xofigo (223Radium chloride) is a predominantly alpha-emitting isotope developed initially at the Radium Hospital in Oslo and then commercially by Algeta and Bayer to treat skeletal metastases in CRPC. Because it has the same physicochemical properties as calcium, it is taken up preferentially into areas of high osteoblastic activity in the bone, which is where the bone metastases are: the metastases cause intense destruction (osteoclastic lysis) of bone, which is met with a bone-forming (osteoblastic) response.
Once in the metastases, 223Ra emits four alpha particles (Fig 1), which cause high levels of tumour cell killing locally [1]. Because of the short path length of the particles, the bone marrow is relatively spared, and high therapeutic ratios are achieved. Off-target effects are limited as the daughter nucleides are excreted through the hepatobiliary system and GI tract, where they cause little toxicity because of the short path length of the alpha particles.
In a randomised Phase 3 study in late-stage CRPC (ALSYMPCA) in patients with bone metastases and no visceral metastases, 223Ra significantly prolonged overall survival compared to placebo, as seen in Fig 3 [4]. This was the first time a radiopharmaceutical had shown survival improvement in a large study of late-stage cancer. Xofigo was registered in the US in 2013 and worldwide in 2014-2016, achieving widespread clinical usage. This clinical and first commercial breakthrough for radiopharmaceutical led to a broad effort to identify alpha and beta emitters, which could be linked to targeting ligands to treat a variety of cancers. Several small companies and some larger ones are now engaged in this effort.
The main limitation of 223Ra is that it only targets and treats osseous secondaries, so patients with visceral metastases need alternative therapies. It is not possible chemically to link 223Ra to a linker molecule.
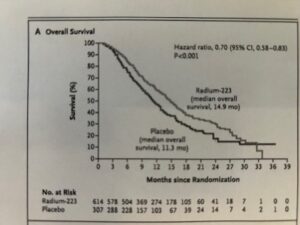
Pluvicto
PSMA is upregulated in prostate cancer and is expressed in prostate, salivary, CNS, duodenal and renal cells. It is a validated prostate cancer target, and the PSMA receptor’s avidity determines efficacy. Pluvicto (177Lutetium-PSMA-617) consists of a small molecule ligand (PSMA-617) chelated to a beta-emitting isotope, 177Lutetium.
In a phase 3 study in late-stage, post-chemotherapy CRPC (VISION), Pluvicto showed significant prolongation of survival in patients whose tumours were positive on 68Gallium-PSMA-PET scanning [5]. In another randomised phase 3 trial, reported at ESMO in November 2023 (PSMAFore), Pluvicto was compared to the standard of care in post-ARPI PSMA-positive patients who had not received chemotherapy. Radiological progression-free survival was significantly improved, and overall survival was longer but did not reach statistical significance because of high (and permitted) crossover to Pluvicto after the treatment period.
Several other PSMA-targeted radiopharmaceuticals are in development, and two phase 3 trials are running: ECLIPSE (by POINT biopharma) and SPLASH (by Curium). Both these agents use 177Lu as the isotope and different PSMA ligands. Other research underway includes 67Cu-PSMA and 67Cu-bombesin targeted agents sponsored by Clarity Pharmaceuticals: these projects are differentiated by the use of a bisPSMA ligand, with higher avidity for the PSMA receptor, and the novel use of 64Cu-bisPSMA for diagnosis and 67Cu-bisPSMA for therapy, which as isotopes have some theoretical advantages for imaging and therapy over 68Ga and 177Lu respectively.
Somatostatin targeted radiopharmaceuticals
Gastro-entero-pancreatic neuroendocrine tumours, or GEP-NETs (formerly called carcinoid), are rare tumours with an incidence of approximately 18,000 patients annually in the United States. Somatostatin receptor type 2 (SSTR2) is expressed in 80-90% of GEP-NET tumours. Tumours can be aggressive and resistant to therapy, with metastatic disease present at diagnosis in 40-76% of cases. Radiopharmaceutical targeted therapy comprising dotatate, a peptide somatostatin analogue with a DOTA chelator bound to 177Lutetium, has shown significant clinical benefit and is approved for the treatment of adult patients with SSTR+ GEP-NETs. Some meningiomas and neuroblastomas also overexpress SSTR2.
Despite the rarity of these conditions, several companies are pursuing new SSTR2-targeted agents. Rayze-Bio is developing an SSTR2 targeting agent using dotatate linked to 225Actinium, an alpha-emitting isotope which decays to 223Ra [6]; Bristol-Myers Squibb has recently acquired the company. Broader interest in Actinium was stimulated by the success of Xofigo, as Actinium, unlike 223Ra, can be chelated to targeted antibodies, peptides and small molecules.
Several targeted alpha therapies (TAT) are in development by several companies, using alpha-emitting isotopes including 225Ac, 211At (Astatin), 212Bi, 212Pb/213Bi, 224Ra, 223Ra, and 227Th [7]. One of the companies is Fusion Pharma, which has an 225 Ac-linked IGF1R targeted product in development and a PSMA-linked product for prostate cancer [8]. Clarity Pharma also has an SSTR2 targeted agent in development for GEP-NET and neuroblastoma, using 64Cu for imaging and 67Cu for therapy [9]. Unlike 223Ra, 225Ac is excreted via the kidney, which is susceptible to alpha radiation, so study protocols include careful examination for late-stage renal toxicity and dosimetry considerations to limit renal exposure.
A new approach: theranostics
Most of the currently targeted radiopharmaceuticals take a so-called theranostic approach, in which PET scanning using the same ligand (e.g. PSMA or SSTR2) linked to a diagnostic radiopharmaceutical (with much lower energy emission, suitable for PET imaging) such as 68Ga or 64Cu, is performed before treatment; if tumour uptake meets the criteria for positivity, treatment with the targeted radiopharmaceutical using the same ligand follows.
Diagnostic imaging determines whether the tumour expresses the antigen to which the ligand is targeted and can be used to assess the extent of the disease. In addition, the radio-diagnostic can be used to assess the effect of the radio-therapeutic and determine whether the residual tumour would benefit from further cycles of therapy. This not only acts as a diagnostic for the tumour but also increases the likelihood of a response. For example, in the Pluvicto trial, using 177Lu-PSMA-617, positive PET imaging with 6868 Ga-PSMA-617 was an entry criterion. Testing for tumour antigen expression has, of course, been around for years – for example, tumour Her2 expression before treatment of Her2+ breast cancer with Herceptin – but in this field, the approach is more or less routine now and does not require tissue. The theranostic approach has not replaced conventional imaging with MRI and CT regarding tumour response assessment. Still, it may well do so in future and is already being used to increase the sensitivity of screening and the likelihood of response by using appropriately targeted radiotherapeutics.
Summary
Following the success of the alpha-emitting 223RaCl2 (Xofigo) in prostate cancer, research and investor interest have been kindled in the further development of targeted radiopharmaceuticals in oncology, with a theranostic approach often being used. The field is growing fast, and many of these agents will come into clinical use in the next decade, further increasing the options for patients with difficult-to-treat and late-stage tumours.
Pluvicto and Xofigo in prostate cancer and SSTR2 targeting agents in GEP-NET tumours are already in clinical use and offer significant benefits in these conditions. Provided the toxicity liabilities can be addressed and are not overly limiting, the superior therapeutic ratio achievable with alpha emitters leads me to believe that this class will achieve greater prominence as the pipeline of agents matures in the coming decade.
Conflicts of Interest
Dr O’Bryan-Tear is the former Chief Medical Officer of Algeta and a former non-executive director at Clarity Pharmaceuticals.
Dr C Gillies O’Bryan-Tear MB FRCP FFPM MBA
Dr O’Bryan-Tear obtained his MD degree from the Universities of Cambridge and London in the UK and trained in internal medicine in the UK NHS. He joined the pharmaceutical industry in 1986, where he has spent 30 years in clinical development, medical management and commercial roles. He was Medical Director, UK and Northern Europe for Bristol-Myers Squibb 1996-2002, where he was responsible for the clinical development of all BMS compounds in the UK and Northern Europe. Dr O’Bryan-Tear then became VP and head of Clinical Development for GlaxoSmithKline Biologicals, where he had global responsibility for the clinical development of GSK vaccines. From 2003 to 2009, he ran his own business, providing clinical development and management consultancy to a broad range of small and large R&D-based pharmaceutical companies. In 2009, he became Chief Medical Officer of Algeta AS, a listed Norwegian biotech company developing a product for prostate cancer. Following the worldwide launch of this product in 2013, the company was sold to Bayer AG for $2.9bn. Since then, Dr O’Bryan-Tear has sat on the Scientific Advisory Boards of several US and EU biotech companies and the Board of Clarity Pharmaceuticals in Australia.
Fellow of the Faculty of Pharmaceutical Medicine, UK; Past Chair of the Policy and Communications Group, Faculty of Pharmaceutical Medicine; Fellow, Royal College of Physicians; MBA, Cranfield Business School, UK.
References
[1] Howell, R.W., Goddu, S.M., Narra, V.R., Fisher, D.R., Schenter, R.E. and Rao, D.V. (1997). Radiotoxicity of Gadolinium-148 and Radium-223 in Mouse Testes: Relative Biological Effectiveness of Alpha-Particle Emitters In Vivo. Radiation Research, 147(3), p.342. doi:https://doi.org/10.2307/3579342.
[2] Algeta ASA (2013). Penetration of radiation.
[3] Tannock, I.F., de Wit, R., Berry, W.R., Horti, J., Pluzanska, A., Chi, K.N., Oudard, S., Théodore, C., James, N.D., Turesson, I., Rosenthal, M.A. and Eisenberger, M.A. (2004). Docetaxel plus Prednisone or Mitoxantrone plus Prednisone for Advanced Prostate Cancer. New England Journal of Medicine, 351(15), pp.1502–1512. doi:https://doi.org/10.1056/nejmoa040720.
[4] Parker, C., Nilsson, S., Heinrich, D., Helle, S.I., O’Sullivan, J.M., Fosså, S.D., Chodacki, A., Wiechno, P., Logue, J., Seke, M., Widmark, A., Johannessen, D.C., Hoskin, P., Bottomley, D., James, N.D., Solberg, A., Syndikus, I., Kliment, J., Wedel, S. and Boehmer, S. (2013). Alpha Emitter Radium-223 and Survival in Metastatic Prostate Cancer. New England Journal of Medicine, 369(3), pp.213–223. doi:https://doi.org/10.1056/nejmoa1213755.
[5] Nitin Vaishampayan, Morris, M.A., Krause, B.J., Vogelzang, N.J., Ayse Tuba Kendi, Nordquist, L.T., Calais, J., Nagarajah, J., Beer, T.M., Ghassan El-Haddad, Brackman, M.A., DeSilvio, M., Messmann, R.A., Sartor, O. and Karim Fizazi (2022). [177Lu]Lu-PSMA-617 in PSMA-positive metastatic castration-resistant prostate cancer: Prior and concomitant treatment subgroup analyses of the VISION trial.. 40(16_suppl), pp.5001–5001. doi:https://doi.org/10.1200/jco.2022.40.16_suppl.5001.
[6] RayzeBio. (n.d.). Front Page. [online] Available at: https://rayzebio.com/.
[7] Bruland, Ø.S., Larsen, R.H., Baum, R.P. and Juzeniene, A. (2023). Editorial: Targeted alpha particle therapy in oncology. Frontiers in Medicine, [online] 10, p.1165747. doi:https://doi.org/10.3389/fmed.2023.1165747.
[8] Fusion Pharma. (n.d.). The Evolution of Radiopharmaceuticals – Fusion Pharma – Home. [online] Available at: https://fusionpharma.com/.
[9] Clarity Pharmaceuticals – Radiopharmaceutical Therapies and Imaging. (n.d.). Clarity Pharmaceuticals – Radiopharmaceutical Therapies and Diagnostics. [online] Available at: https://www.claritypharmaceuticals.com/.