Inhalation therapies for COVID-19
Posted on: Thursday 28 January 2021
Author: Bob Humphries et al.
This article has been prepared by Bob Humphries, Prof Dr. H.W. Frijlink, Reinier Schwietert, PhD and Prof Tim Higenbottam.
It is provided for information and does not constitute advice or represent official FPM views or policy.
How to cite:
Humphries, B. et al (2020), ‘Inhalation therapies for COVID-19’, Faculty of Pharmaceutical Medicine blog, 28 January 2021. Available at: https://www.fpm.org.uk/blog/inhalation-therapies-for-covid-19/ (Accessed: <date>).
Introduction
The means to control the SARS-CoV-2 virus pandemic is now emerging, with the recent approval of the RNA vaccine from Pfizer/BioNTech1 and the COVID-19 Vaccine (ChAdOx1 S [recombinant])2. Both vaccines have been shown to be free of serious adverse events and have significant efficacy in creating immunity to the current virus. A new B.1.1.7 variant with substantially higher transmissibility than previous SARS-CoV-2 viruses has emerged in the UK recently. It is still believed to be affected by the immunological response to the new vaccines. It is more easily transmitted with an increased R0 number from 2.5 by 70% or more3 compared with the earlier variant. Emergence of a SARS-CoV-2 variant that is unresponsive to the current vaccines is becoming a possibility. Furthermore, the virus may persist in the respiratory tract even with established immunity from a prior infection, leaving the potential for further transmission4.
The commentary and review are written to encourage the development of community based inhaled anti-viral therapy to inhibit the initial stages of the infection of the respiratory tract, not as an alternative to the vaccine programme but to manage the future risk of a resistant variant SARS-CoV-2 or new SARS viruses.
The clearly separate phases of the COVID-19 disease
COVID-19 disease follows a phasic pattern, with different disease mechanisms driving pathology in each phase of the illness. The early phase starts when the virus has entered the respiratory tract epithelial cells. During this phase, in which affected individuals may shed virus laden aerosols and thus may infect contacts, viral load in the respiratory tract rapidly increases, reaching a peak within 7 – 12 days following infection, after which time the viral load and infectivity rapidly decreases3. Treating people with an effective antiviral in this phase of the illness could not only prevent the latter stages of COVID—19 disease in the individual, but potentially might limit disease spread.
The second phase of COVID-19 disease is associated with increasing breathlessness and hypoxia with radiological changes seen in the chest X-ray5. This phase of the illness is the result of immune dysregulation. Many patients in this phase of disease require ventilation and may progress to other more complicated multiorgan system involvement with a high fatality rate6.
Figure 1: The stages of the COVID-19 disease7
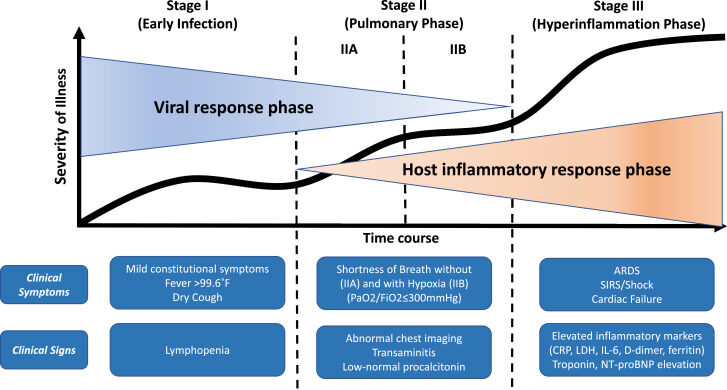
The need to identify medicines that can be delivered in the community to control the infection
Community-based interventions are urgently needed to stop viral replication and reduce risk of disease progression and viral spreading. Such a treatment should be safe and suitable for widespread use. Studies with such medicines should be undertaken in the community, where most of the infected patients are found and where the infection is spread. Despite the arrival of several effective vaccines, continued spread of the virus within the community requires urgent attention to the production of effective antiviral medicines suitable for community use to control disease in the early phase of viral replication and prevent the need for hospitalisation of affected individuals.
Here we would like to consider treatments that could reduce viral entry and/or halt viral replication as rapidly as possible. Regrettably, currently recognised antiviral treatment has predominantly been investigated in patients admitted to hospital. However, at this point in the illness, viral load is already declining, and the disease pathology is driven by immune dysregulation and coagulopathy, which cannot be expected to respond to antiviral medication.
Following this proposition, patients with symptoms suggestive of COVID-19 disease should be treated as rapidly as possible, potentially starting before diagnostic confirmation. Chemo prophylaxis could also be considered for all close contacts of a potentially infected person. If successful, both posologies would be expected to significantly reduce the consequences of the infection in the community and would have the added benefit of limiting the spread of disease.
The challenges of early treatment of COVID-19 - consideration of inhalation therapy
In attempting to prevent or treat early SARS-CoV-2 infections, the imperative is to target the “port of entry” of the virus – the respiratory tract and airway epithelium. To be maximally effective, this targeting must be rapid and of high intensity, aiming to nullify viral entry and replication before it becomes established. Success in this regard will be dependent on application of 2 fundamental PK/PD principles: –
- Concentrations of the anti-viral at the site of action (the airway epithelium) are more relevant than plasma concentrations to predict efficacy
- The target concentration for complete viral suppression should be based on the EC90/95 for inhibition of viral replication
These principles are re-iterated and expanded upon in the individual examples discussed below.
Figure 2: Respiratory Tract as the entry site for SARS-CoV-2
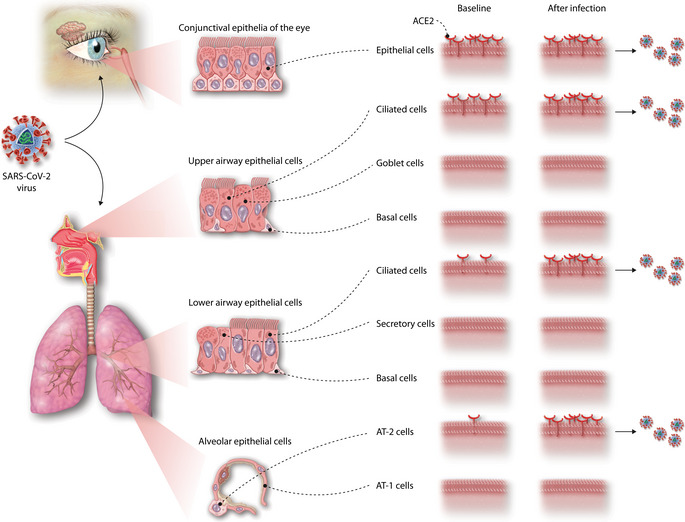
Although treatment with an oral tablet is simple, it can be challenging to rapidly achieve high airway drug exposure via this route. In addition, the high systemic exposure required to drive the required airway exposure may limit widespread use because of safety issues.
This situation is not unprecedented. There are many examples in which the clinical imperative has been to achieve efficacious concentrations of a drug rapidly and safely in the airway, but where this has been challenging with oral dosing due to systemic side effects or absorption and distribution issues. In such cases, inhaled delivery can and has provided the solution, for example inhalational aminoglycosides, pentamidine and ribavirin.
Figure 3: The Inspired Choice: Delivering to the Airway for the Airway
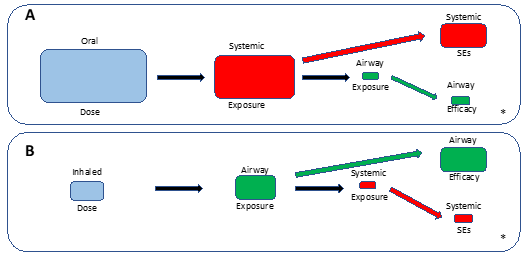
With inhaled delivery (B), airway exposure and efficacy is achieved with a lower total dosage, lower systemic exposure and reduced systemic side effect – high airway exposure is achieved instantaneously leading to immediate onset of effect.
*Relative proportions are illustrative and will be different for different agents depending on dose, bioavailability and pharmacokinetic properties
Repurposing existing medicines for inhalation is not straightforward, with a significant amount of re-formulation, device development and optimisation required. However, multiple options are available, including various nebuliser-based approaches, and dry powder inhalers (DPIs). Furthermore, the alteration of the route of administration calls for a thorough evaluation and investigation of pharmacokinetics and local safety.
On the other hand, a particular value of inhaled repurposing of a drug originally formulated for oral (or parenteral) administration is that the total dose can be reduced. This is exemplified by several examples now in clinical trials or being prepared for first into human studies in SARS-CoV-2 infection.
Drug | Current Dose & Route | Inhaled Dose & Formulation/Device | Status (latest phase completed) | Code/Developer |
---|---|---|---|---|
Remdesivir | 100-200 mg (iv) | 31, 62 mg (nebulised) | Phase I | Gilead |
Hydroxychloroquine | 800 mg loading then 400 mg/day (oral) | 4, 8, 12 mg (nebulised) | Phase I | TLC19 |
Hydroxychloroquine | As above | 20 mg (dry powder) | Phase I | Cyclops HCQ/PureIMS |
Hydroxychloroquine | As above | Upto 50 mg (nebulised) | Phase I | Pulmoquine |
IFN-β1a (SNG001) | Adults, dose of Avonex is 30 micrograms, given by injection into a muscle once a week Rebif 44 mcg of interferon beta-1a administered by a 0.5 mL subcutaneous injection on Days 1, 3, 5, and 7 while hospitalized for a total of 4 doses National Institute of Allergy and Infectious Diseases (NIAID) | 6 mIU of IFN-β | Phase II | SNG001/Synairgen |
Niclosamide | 2 gm as a single dose | Not yet released | Phase I | UNION Therapeutics |
PUL-042 | N/A | Solution 20.3 µg Pam2: 29.8 µg ODN/mL (50 µg PUL-042) | Phase I | Pulmotect |
Remdesivir: from intravenous to inhaled administration
Remdesivir is the only currently approved anti SARS-CoV-2 antiviral. Its use is limited to hospitalised patients, as it is currently available only as an IV preparation8. Remdesivir is the pro-drug of the effective nucleotide triphosphate antiviral, which is produced intracellularly in vivo. The cellular concentrations of the triphosphate required to inhibit SARS CoV2 replication in the lung are higher than may be achieved using the current IV dose regimen9.
Gilead have now followed early suggestions that inhaled remdesivir should be more effective than oral10. They have begun a study of nebulised remdesivir in COVID-19 patients. Delivering remdesivir directly to the primary site of infection with a nebulized, inhaled solution may enable more targeted and accessible administration in non-hospitalized patients. This US-based trial will include around 60 healthy adults aged 18-45 and will first test how patients react to the inhaled solution. If the first study is successful, nebulised remdesivir will be rolled out across outpatient services – such as GP surgeries – to medicate patients who do not need hospital treatment.
In addition, University of Texas, Austin/TFF Pharmaceuticals is exploring a dry powder inhaled formulation of remdesivir11. The inhaled formulation could deliver patients a lower-cost, lower-dose version of the drug that tackles the disease in its early stages. The thin film freezing technology prevents drug degradation and cuts out the need to use large amounts of excipients, so the final product can have a high remdesivir content.
These observations on inhaled remdesivir demonstrate potential means of treating infected COVID-19 patients with anti-viral agents as early as possible to limit the symptoms and to prevent progression in high-risk patients. These clinical trials will need to be undertaken in the community settings.
Hydroxychloroquine: from oral to inhaled administration
In the early months of the COVID-19 pandemic, the repurposing candidate investigated in many of the early trials was orally administered hydroxychloroquine (HCQ), which is used to treat malaria and intractable autoimmune disease. Following a large number of unsuccessful trials with oral HCQ in hospitalised and community-based patients, further trials were abandoned. The doses of HCQ used in these trials were unlikely to have achieved adequate concentrations in the lung epithelium. The proposed mechanism of action of HCQ in COVID-19 is to reduce viral entry by inhibiting the binding of SARS-CoV-2 spike protein to the epithelial cells ACE2 receptors and to inhibit viral replication through elevation of the endosome pH, so inhibiting virus endosome fusion12,13.
Several groups14 have now subjected the PK/PD of HCQ in more scrutiny, with a consensus now building that, with the oral dosing regimens that can be safely used, the lung airway concentrations of HCQ will be lower than those required to exert an antiviral effect. This shortcoming of oral dosing of HCQ is further exacerbated by the fact that it takes four days to achieve the target exposure, meaning that an important therapeutic window was missed. The use of higher oral doses is not an option. With the doses used in the COVID-19 setting, concerns have already been expressed regarding systemic side effects, in particular the risk of QT prolongation, and there seems to be little scope to increase oral doses without also increasing this risk15.
Therefore, assuming the target for HCQ is in the lung, rigorous PK/PD modelling tells us that the “failure” of the studies conducted to date with systemic HCQ were inevitable, reflecting a lack of alignment between the anti-viral mechanism of HCQ, its PK/PD properties (particularly airway exposure following oral dosing) and the stage of COVID-19 in which the studies were conducted. Crucially, the required clinical exposure assumed that a target concentration equivalent to or just above the EC50 for inhibition of viral replication by HCQ in vitro would be sufficient for efficacy. However, it is evident from studies with other viruses and anti-virals that complete viral suppression requires a higher concentration (EC95)16. Inhalation can provide the solution.
Figure 4: Achieving local high concentration of the anti-viral early in the infection process
There are now 3 inhaled formulations of HCQ ready for or already in clinical studies. In 2005 Aradigm/APT Pharmaceuticals took a nebulised formulation of HCQ into a PhII asthma study using the Aradigm AERx soft mist device17. This involved dosing of approximately 100 patients for up to 21 days, establishing the inherent safety of HCQ delivered by nebulisation. A formulation based on this experience has now been evaluated by Pulmoquine in a Phase 1 study in the US18. In the Netherlands, PureIMS, in collaboration with the University Medical Centre Groningen, University of Groningen, and several international partners, has completed a first in human trial using a dry powder formulation of HCQ in the PureIMS Cyclops™ inhaler19. Most of the studies conducted with oral HCQ have used a loading dose of 800 mg followed by twice daily doses of 400 mg. The intended inhaled dose from the Cyclops™ inhaler to achieve high local concentrations in the airway is just 20 mg.
Video 1: The Cyclops dry powder inhaler
Inexpensive single dose dry powder inhaled, avoids contamination and provide excellent lung distribution19.
Finally, TLC have recently initiated Phase 1 evaluation of an inhaled liposomal formulation of HCQ (TLC19) administered using a portable vibrating mesh nebuliser20.
These 3 initiatives will quickly determine whether inhaled HCQ (iHCQ) can have a significant effect on viral entry and/or load in the airway. This should lead to less transmission of virus particles early in disease, limiting the spread of infection. Inhalation offers the real possibility that better efficacy can be achieved more rapidly and with a lower total dose. A simple dry powder formulation of HCQ in an easy-to-use device would be a credible means to provide widespread deployment of an accessible, affordable early treatment/preventative for COVID-19.
Niclosamide: from oral to inhaled administration
Niclosamide is used for the treatment of tapeworm infections. It is taken by mouth and is not absorbed, working by inhibiting anaerobic metabolism in the tapeworm. It is a WHO listed essential medicine.
It has been known that Middle East respiratory syndrome coronavirus (MERS-CoV) multiplication results in reduced Beclin1 (BECN1) levels and blocks the fusion of autophagosomes and lysosomes. Autophagy is an essential cellular process for control of virus infections, and BECN1 is one of its key regulators. The S-phase kinase-associated protein 2 (SKP2) executes lysine-48-linked poly-ubiquitination of BECN1 which promotes proteasomal degradation. Pharmacological inhibition of SKP2 decreases BECN1 ubiquitination, decreases BECN1 degradation and enhances autophagic flux. Inhibitors of SKP2 not only enhance autophagy but also reduce the replication of MERS-CoV up to 28,000-fold21. The SKP2-BECN1 link constitutes a promising target for host directed antiviral drugs and possibly other autophagy-sensitive conditions.
Niclosamide inhibits MERS-CoV replication by up to 1000-fold at 48 h p.i. at a concentration of 10 μM, while it enhanced the BECN1 level and ATG14 oligomerization, increased the number of autolysosomes by >2-fold, and affected the autophagic flux in the MERS-CoV-infected cells. Since niclosamide is a multifunctional drug, we cannot exclude the possibility that it exerts its anti-MERS activity by regulating other targets besides SKP2 inhibition. NIC-hLYS is effective in vitro and in vivo for the treatment of COVID-19 using a mouse model of MERS and SARS-CoV-2 infection and, importantly, scalable inhaled drug delivery systems can be developed based upon the formulation which will enable rapid availability to a global patient population. The Institute Pasteur Korea, Seongnam, Korea similarly showed that niclosamide compared with 48 FDA approved medicines in vitro was highly effective in inhibiting SARS-CoV-2, some 40 times more potent than remdesivir.
In terms of pharmaceutical development, a major limitation of NIC as an antiviral therapy is its poor solubility in water, which is reported at 1.6 mg/L22. This makes systemic absorption of the drug by the oral route of administration at therapeutically relevant concentrations difficult. Administration of the existing FDA-approved chewable tablet formulation was found to result in inadequate systemic concentrations for inhibiting SARS-CoV-2 replication.
University of Texas, Austin has undertaken significant reformulation of niclosamide making use of human lysozyme (hLYS), an endogenous protein in the upper and lower respiratory tracts, as a therapeutically active-matrix material for the delivery of NIC to the airways, based upon its known anti-inflammatory, antibacterial and antiviral,23,24,25,26, including SARS viruses, as well as its surface-active properties. The antiviral, antibacterial, and anti-inflammatory efficacy of the NIC-hLYS powders were evaluated in vitro and in vivo in MERS-CoV and SARS-CoV-2 infection mouse models. The composition of the NIC-hLYS formulation was optimized using a constrained mixtures Design of Experiments (DoE) approach to achieve particles with a size appropriate for inhalation both in the dry powder state and when reconstituted in aqueous media. Physicochemical characterization of the optimized powder was performed, and nasal, DPI, and nebulizer systems were developed and tested.
Inhibition of the corona viruses was tested with NIC-hLYS particles (0.7% w/w NIC) administered at varying concentrations (based upon NIC content) to Vero E6 cells infected with MERS-CoV or SARS-CoV-2, and the EC50 was calculated based upon observed cytopathic effect (CPE). The addition of hLYS to the NIC formulation resulted in improved antiviral activity based upon reductions in the EC50 dose for MERS-CoV (0.016 μg/mL NIC to 0.0625 μg/mL NIC) and SARS-CoV-2 (0.030 μg/mL to 0.008 μg/mL).
The Danish biotech company UNION Therapeutics Ltd have undertaken a successful multiple dose nebulised niclosamide study using a proprietary salt solution in normal volunteers completed in July 2020. In August they signed an agreement with TFF Pharmaceuticals who specialise in thin film formulation to enable dry powder formulation for inhalation. They have approval to start a trial in COVID-19 patients.
Interferon-b1a SNG001: from Intra-muscular (i.m.) or subcutaneous (s.c.) to inhaled administration
Interferons are cytokines that modulate immune responses to viral infection. The type I interferons (interferon alphas and interferon beta [IFN-β]) have been tested against coronavirus infections in vitro with encouraging results. IFN-β-mediated anti-viral responses have been shown to be compromised in people susceptible to COVID-19, such as older people or patients with chronic airway diseases. Furthermore, SARS-CoV-2 suppresses cellular IFN production, limiting the strength of the initial innate immune response27. This could be of particular importance in limiting the immune dysregulation that causes COVID-19 pneumonia.
IFN- β1 administered i.m. or s.c. is an approved treatment for relapsing multiple sclerosis. Exogenous use of inhaled IFN-β1a (SNG001) delivered by a nebuliser has already been tested in patients with asthma with respiratory viral infections. It reduces symptomatic responses to the virus infection and improves lung function. Similar results have been reported in exacerbating COPD patients. These properties may facilitate faster improvement and/or recovery in patients with COVID-19. A clinical study has just been completed to evaluate the potential effects of an inhaled IFN-β1a formulation in patients hospitalised with confirmed SARS-CoV-2 infection.
In this study28, using the I-neb delivery platform, 101 patients were randomised; 48 received SNG001 and 50 received placebo (ITT population). Patients who received SNG001 had greater odds of improvement across the OSCI scale (OR 2·32; 95% CI: 1·07, 5·04; p=0·033) and were more likely to recover to “no limitation of activity” during treatment (HR 2·19; 95% CI: 1·03, 4·69; p=0·043). SNG001 reduced patient reported BCSS symptom score (difference -0·8, 95% CI: -1·5, -0·1; p=0·026), mainly by lowering the breathlessness score (difference -0·6, 95% CI: -1·0, -0·2; p=0·007). There were three deaths in the placebo and none in the SNG001 groups. SNG001 was well-tolerated and patients recovered more rapidly, providing a strong rationale for further trials.
The follow-on, home-based part of the study29 is now ongoing. This part will be conducted in the patients’ homes and will target an at-risk patient population (elderly and/or patients with co-morbidities). This should allow the assessment of both home delivery of SNG001 and of the safety/efficacy at an early stage of the disease. This study is using the Aerogen Solo/Ultra nebuliser, which will also be used in the phase 3 study. The Aerogen Solo is a closed-system, single-patient use (vibrating mesh) aerosol drug delivery technology, which the company claims to offer superior performance across all hospital ventilation modalities. A closed-circuit design reduces the risk to others of exposure to bioaerosols.
Figure 5: Aerogen Solo is a closed-system, single-patient use (vibrating mesh) aerosol drug delivery technology
Inhaled Pulmotect 042 (PUL-042)
Respiratory viruses are detected by Toll-like receptors (TLRs), which are highly conserved pattern-recognition receptors on respiratory epithelia, smooth muscle, and inflammatory cells30, 31,32,33. The detection by TLRs of molecular motifs on invading microorganisms triggers immune responses, including the production of interferons, cytokines, and antimicrobial factors via the activation of NF-kB (T40). Defective TLR signalling and TLR polymorphisms have been associated with increased susceptibility to infection.
Concurrent treatment with the synthetic TLR2/6 lipopeptide agonist Pam2CSK4 (Pam2) and the Class C oligodeoxynucleotide TLR9 agonist ODN2395 (ODN) was found to reduce lethality and the microbial burden in murine models of bacterial and viral pneumonia34,35,36,37. Indeed, simultaneous treatment with Pam2 and ODN, administered 24 hours before infection, inhibited parainfluenza virus replication and virus induced AHR in a guinea pig model of viral pneumonia in vivo. In mouse models, a single inhaled dose of PUL-042 was shown to protect the host from SARS-CoV, and the drug significantly reduced the amount of virus in the lungs after infection with either the SARS-CoV or MERS-CoV viruses. PUL-042 combines Pam2 and ODN and has now entered trials for the treatment of COVID-19.
There are two phase 2 clinical trials in COVID-19 with PUL-042, delivered using PARI’s LC Sprint Reusable Nebulizer. Study NCT0431299738 started in June 2020 in 100 patients, to determine the efficacy of PUL-042 Inhalation Solution in decreasing the severity of COVID-19 with documented SARS-CoV-2 infection and not requiring supplemental oxygen (Ordinal Scale for Clinical Improvement (OSCI) 3 or less) at the time of enrolment. The primary endpoint is the difference in the proportion of patients with clinically meaningful worsening of COVID-19 within 28 days from the start of experimental therapy, as indicated by an increase of at least 2 points on the OSCI. The second study (NCT04313023)39 began in June 2020 in 200 patients who have repeated exposure to individuals with SARS-CoV-2 infection and are asymptomatic at enrolment. The primary endpoint is the severity of COVID-19 as measured by the maximum difference from the baseline value in the Ordinal Scale for Symptom Improvement within 28 days from the start of experimental therapy. These patients will be treated at home.
In Conclusion
History tells us inhaled therapies can be extremely successful. Beclomethasone dipropionate (BDP) was patented in 1962. It was initially used topically as a cream applied for skin rashes. However, the value of local administration was not initially appreciated for asthma and it took 10 years to be delivered in an inhaler. It proved to one of the most successful of medicines. Inhaled in a small dose it was possible to take asthma patients off oral or intravenous steroids and still control the illness. It is on the World Health Organization’s List of Essential Medicines. Today many inhaled corticosteroids have followed the beclomethasone example.
While it remains to be seen which of the potential inhaled approaches outlined demonstrate the most attractive efficacy/safety profile, other factors will also need to be considered not least the cost of treatment. A 5-day course of intravenous remdesivir is £1900 in the UK. Affordability is important when considering early treatment/prevention in a large proportion of the population. A simple dry powder formulation of a generic drug in an easy-to-use device would be a credible means to provide widespread deployment of an accessible, affordable early treatment/preventative for COVID-19.
LESSONS on repurposing existing medicines:
- Understand the disease and define exactly the purpose that the repurposed medicine is required to achieve
- Test the medicine preclinically to define the exact dose of the medicine using in vitro and in vivo studies – base this on stringent application of PK/PD principles, particularly the need to achieve adequate exposure (EC90/95) at the target site
- Study patients in small numbers first as you would for a first in human study. Safety is the main concern and the disease process may alter the recognised pharmacokinetics and pharmacodynamics
- The proof of efficacy and safety requires randomised controlled studies – where the choice of the primary outcome measurement is tightly linked to the purpose proposed for the medicine
- For lung disease always consider an inhaled route of delivery to reduce the total body dose and to concentrate the medicine on the lung tissue and respiratory tract – reformulate if necessary
References
- European Centre for Disease Prevention and Control. Rapid increase of a SARS-CoV-2 variant with multiple spike protein mutations observed in the United Kingdom – 20 December 2020. ECDC: Stockholm; 2020
- What Pfizer’s landmark COVID vaccine results mean for the pandemic. Nature-on-line. https://www.nature.com/articles/d41586-020-03166-8.
- Voysey M, Costa Clemens SA, Madhi SA, et al. Safety and efficacy of the ChAdOx1 nCoV-19 vaccine (AZD1222) against SARS-CoV-2: an interim analysis of four randomised controlled trials in Brazil, South Africa, and the UK. Lancet – Published Online December 8, 2020 https://doi.org/10.1016/ S0140-6736(20)32661-1
- Hopkins S. Press release: Past COVID-19 infection provides some immunity, but people may still carry and transmit virus. Public Health England published 14th January 2021
- Huang C, Wang Y, Li X, et al. Clinical features of patients infected with 2019 novel coronavirus in Wuhan, China. Lancet. 2020 Feb;395:497–506. doi:10.1016/S0140-6736(20)30183-5external icon
- ICNARC report on COVID-19 in critical care: England, Wales and Northern Ireland 27 November 2020. https://www.icnarc.org/Our-Audit/Audits/Cmp/Reports.
- Siddiqi H K, Mehra M R. COVID-19 illness in native and immunosuppressed states: A clinical-therapeutic staging proposal. J Heart Lung Transplant. 2020; 39:405-407. doi: 10.1016/j.healun.2020.03.012.
- Brown AJ, Won JJ, Graham RL, et al. Broad spectrum antiviral remdesivir inhibits human endemic and zoonotic delta coronaviruses with a highly divergent RNA dependent RNA polymerase. Antiviral Res 2019; 169: 104541.
- Beigel JH, Tomashek KM, Dodd LE. Remdesivir for the Treatment of Covid-19 — Final Report N Engl J Med 2020; 383: 1813-26. DOI: 10.1056/NEJMoa2007764
- Sun D. Remdesivir for Treatment of COVID-19: Combination of Pulmonary and IV. American Association of Pharmaceutical Scientists Published on-line 2020 April. DOI: 10.1208/s12248-020-00459
- Sahakijpijarn S, Moon C, Koleng JJ, et al., Development of Remdesivir as a Dry Powder for 1 Inhalation by Thin Film Freezing. This version posted September 22, 2020; doi: https://doi.org/10.1101/2020.07.26.222109 bioRxiv preprint
- M.J. Vincent et al., “Chloroquine is a potent inhibitor of SARS coronavirus infection and spread,” Virol. J., vol. 2, pp. 1–10, 2005, doi: 10.1186/1743-422X-2-69.
- J. Liu et al., “Hydroxychloroquine, a less toxic derivative of chloroquine, is effective in inhibiting SARS-CoV-2 infection in vitro,” Cell Discov., vol. 6, no. 1, pp. 6–9, 2020, doi: 10.1038/s41421-020-0156-0.
- Fan J, Zhang X, Liu J, et al., “Connecting Hydroxychloroquine In Vitro Antiviral Activity to In Vivo Concentration for Prediction of Antiviral Effect: A Critical Step in Treating Patients With Coronavirus Disease 2019,” Infect. Dis., 2020, doi: 10.1093/cid/ciaa623.
- M. Garcia-Cremades et al., “Optimizing Hydroxychloroquine Dosing for Patients With COVID-19: An Integrative Modeling Approach for Effective Drug Repurposing,” Clin. Pharmacol. Ther., vol. 108, no. 2, pp. 253–263, 2020, doi: 10.1002/cpt.1856
- G.L. Drusano et al., “Hollow-fiber unit evaluation of a new human immunodeficiency virus type 1 protease inhibitor, BMS-232632, for determination of the linked pharmacodynamic variable,” J. Infect. Dis., vol. 183, no. 7, pp. 1126–1129, 2001, doi: 10.1086/319281
- Kavanagh O, Healy AM, Dayton F, et al., “Inhaled hydroxychloroquine to improve efficacy and reduce harm in the treatment of COVID-19,” Medical Hypotheses. 2020, doi: 10.1016/j.mehy.2020.110110
- https://clinicaltrials.gov/ct2/show/NCT04461353.
- de Reus Y, Hagedoorn P, Sturkenboom M, et al., Tolerability and Pharmacokinetic Evaluation of Inhaled Dry Powder Hydroxychloroquine in Healthy Volunteers, medRxiv preprint doi: https://doi.org/10.1101/2020.12.03.20243162]
- https://www.tlcbio.com/en-global/press-releases/detail/News_20201014.
- Gassen NC, Niemeyer D, Muth D et al. Beclin1-ubiquitination and its inhibition reduces MERS-Coronavirus infection Nature Communications | (2019) 10:5770 | https://doi.org/10.1038/s41467-019-13659-4 nature.com/naturecommunications
- Kang JE, Rhie SJ. Practice considerations on the use of investigational anti-COVID-19 medications: Dosage, administration, and monitoring. Clinical Pharmacy and Therapeutics. 2020; May https://doi.org/10.1111/jcpt.13199
- Xu J, Shi P-Y, Li H, Zhou J. Broad Spectrum Antiviral Agent Niclosamide and Its Therapeutic Potential. ACS Infectious Diseases. 2020. March https://dx.doi.org/10.1021/acsinfecdis.0c00052
- Smyth HDC Inhaled Niclosamide a Potential Effective Antiviral to Treat COVID-19 (https://research.utexas.edu/showcase/college/college-of-pharmacy)
- Niyomdecha N, Suptawiwat O, Boonarkart C, Jitobaom K, Auewarakul P. Inhibition of human immunodeficiency virus type1 by niclosamide through mTORC1 inhibition. Heliyon 2020; 6: 1 -10. https://doi.org/10.1016/j.heliyon.2020.e04050
- Brunaugh AD, Seo H, Warnken Z, Ding L, Seo SH, Smyth HDC. Broad-spectrum, patient-adaptable inhaled Niclosamide-lysozyme particle are efficacious against Coronavirus in lethal murine infection models. October 2020 bioRxiv preprint doi: https://doi.org/10.1101/2020.09.24.310490.
- Lee JS, Shin EC. The type I interferon response in COVID-19: implications for treatment. Nat Rev Immunol. 2020 Oct;20(10):585-586. doi: 10.1038/s41577-020-00429-3.
- Monk PD, Marsden RJ, Tear VJ, Brookes J, Batten TN, Mankowski M, Gabbay FJ, Davies DE, Holgate ST, Ho LP, Clark T, Djukanovic R, Wilkinson TMA; Inhaled Interferon Beta COVID-19 Study Group. Safety and efficacy of inhaled nebulised interferon beta-1a (SNG001) for treatment of SARS-CoV-2 infection: a randomised, double-blind, placebo-controlled, phase 2 trial. Lancet Respir Med. 2020 Nov 12. pii: S2213-2600(20)30511-7. doi: 10.1016/S2213-2600(20)30511-7. [Epub ahead of print].
- Trial of Inhaled Anti-viral (SNG001) for SARS-CoV-2 (COVID-19) Infection. gov Identifier: NCT04385095. Trial of Inhaled Anti-viral (SNG001) for SARS-CoV-2 (COVID-19) Infection – Full Text View – ClinicalTrials.gov.
- Sukkar MB, Xie S, Khorasani NM, Kon OM, Stanbridge R, Issa R, Chung KF. Toll-like receptor 2, 3, and 4 expression and function in human airway smooth muscle. J Allergy Clin Immunol 2006; 118: 641–648.
- Muzio M, Bosisio D, Polentarutti N, D’Amico G, Stoppacciaro A, Mancinelli R, van’t Veer C, Penton-Rol G, Ruco LP, Allavena P, et al. Differential expression and regulation of Toll-like receptors (TLR) in human leukocytes: selective expression of TLR3 in dendritic cells. J Immunol 2000; 164: 5998–6004.
- Juarez E, Nunez C, Sada E, Ellner JJ, Schwander SK, Torres M. Differential expression of Toll-like receptors on human alveolar macrophages and autologous peripheral monocytes. Respir Res 2010;1 1: 2.
- Koller B, Bals R, Roos D, Korting HC, Griese M, Hartl D. Innate immune receptors on neutrophils and their role in chronic lung disease. Eur J Clin Invest 2009; 39: 535–547.
- Wong JP, Christopher ME, Viswanathan S, Karpoff N, Dai X, Das D, Sun LQ, Wang M, Salazar AM. Activation of Toll-like receptor signaling pathway for protection against influenza virus infection. Vaccine 2009; 27: 3481–3483.
- Kumar H, Kawai T, Akira S. Toll-like receptors and innate immunity. Biochem Biophys Res Commun 2009; 388:6 21–625.
- Kawai T, Akira S. The roles of TLRs, RLRs and NLRs in pathogen recognition. Int Immunol 2009; 21: 317–337.
- Bhan U, Lukacs NW, Osterholzer JJ, Newstead MW, Zeng X, Moore TA, McMillan TR, Krieg AM, Akira S, Standiford TJ. TLR9 is required for protective innate immunity in Gram-negative bacterial pneumonia: role of dendritic cells. J Immunol 2007; 179: 3937–3946.
- The Use of PUL-042 Inhalation Solution to Reduce the Severity of COVID-19 in Adults Positive for SARS-CoV-2 ClinicalTrials.gov Identifier: NCT04312997. The Use of PUL-042 Inhalation Solution to Reduce the Severity of COVID-19 in Adults Positive for SARS-CoV-2 Infection – Full Text View – ClinicalTrials.gov
Authors:
Bob Humphries
Pharmaceutical Consultant
Director Project Strategy
TherapeutAix UG
Juttastrasse 18 | 52066 Aachen | Germany
Email: bob@therapeutaix.com
web: www.therapeutaix.com
Disclosures: none
Prof Dr. H.W. Frijlink
Department of Pharmaceutical Technology and Biopharmacy (XB21)
University of Groningen
A.Deusinglaan 1
9713 AV Groningen
The Netherlands
E-mail: h.w.frijlink@rug.nl
Disclosure: The employer of HWF has a license agreement with PureIMS on the Cyclops inhaler
Reinier Schwietert, PhD
PureIMS BV
Chief Scientific Officer
Ceintuurbaan Noord 152 | 9301 NZ | Roden | The Netherlands
Email: rschwietert@pureims.com
Web: www.pureims.com
Disclosure: An employee of PureIMS BV
Prof Tim Higenbottam
President
Faculty of Pharmaceutical Medicine
326a City Road
London, ECIV 2PT
United Kingdom
t.higenbottam@fpm.org.uk
Disclosure: TWH is a member of the data safety management committee for the COVID-19 studies for the Synairgen phase II study